Abstract
Microcirculatory dysfunction is important in different
intestinal pathologies. Therefore, it is essential for adequate
therapeutic strategies to be based on reliable microcirculatory
diagnostics. Intestinal microvascular perfusion is regulated by
an intricate interplay of neuroendocrine, paracrine and mechano-sensory
pathways. While rectal microvascular bed can be readily examined
at the patient*s bedside, microcirculation (visualize
the microcirculation at the bedside,Cerebral
microcirculation,brain
microcirculation)of other parts of the
gut can only be assessed intra-operatively or by means of enterostomies. Changes in intestinal microcirculation in various
diseases, as observed in animal experiments, further contribute
to our understanding of intestinal microcirculation(brain
microcirculation) in humans.
If microcirculatory(brain
microcirculation) changes are not adequately taken care of,
perfusion will be reduced and
tissue oxygenation may be endangered. Relevant clinical studies
are presented in this article. Future developments, e. g.
miniaturization of optical probes or swallow-able cameras, will
facilitate sophisticated diagnostics and thus improve treatment
results.
Key words: microcirculation, intestinal, imaging,
microcirculatory perfusion
Introduction
All organs are dependent on microvascular perfusion to function
and survive, because individual cells need supply of oxygen and
nutrients and elimination of waste products via the
microcirculation (1)(visualize the
microcirculation at the bedside,Cerebral
microcirculation,brain
microcirculation). In clinical practice,blood pressure and
cardiac output measurements are used for circulatory
assessments, but central hemodynamic parameters can be
satisfactory in spite of critical insufficiency at the
microcirculatory level (2).
Sophisticated techniques are required for assessment of
microcirculation(brain
microcirculation). With regard to the intestinal microcirculation
(IMIC)(visualize the microcirculation at
the bedside,Cerebral microcirculation,brain
microcirculation), only the rectal microvascular bed is readily available
for human examination at the bedside.The microcirculation of
other parts of the gut can only be assessed intra-operatively or
via examination of preexisting enterostomies, respectively.
In the present review we summarize intestinal microcirculatory
(brain
microcirculation)changes in various diseases that can be observed in animal
experiments, and provide an overview on the current status of
intestinal microcirculation(brain
microcirculation) diagnostics in humans. Furthermore,
we screened the available literature regarding clinical imaging
studies of the IMIC. Finally, we discuss requirements for future
developments in intestinal microcirculation(brain
microcirculation) research.
Intestinal microcirculatory changes in various diseases
Intestinal microvascular perfusion is regulated by an
intricate interplay of neuroendocrine, paracrine, and mechano-sensory
pathways. These mechanisms adapt to the balance between
locoregional tissue oxygen transport and metabolic needs to
ensure that supply matches demand (3).
Animal experiments have revealed intestinal microcirculatory
dysfunction in various diseases. Most drastical changes are
observed in sepsis. The gastrointestinal tract plays an
important role in sepsis, since disturbances of the intestinal
microcirculation (visualize the
microcirculation at the bedside,Cerebral
microcirculation,brain
microcirculation)can lead to disruption of the mucosa barrier
and result in bacterial and toxin translocation into the
systemic circulation (gut motor hypothesis,(4)). Several changes
have been described within the microcirculation, such as
decreased deformability of red blood cells due to an increase in
membrane lipid viscosity (5, 6), an increased percentage of
activated neutrophils with decreased deformability and increased
aggregability due to upregulation of adhesion molecules (6, 7),
activation of the clotting cascade with fibrin deposition and
the formation of microthrombi (8, 9), and secondary enhanced
perfusion of large arteriovenous shunts (10). A characteristic
phenomenon of intestinal microcirculatory disturbances in sepsis
is the heterogeneity of the changes with some capillaries being
normally or even hyper-perfused while others are hypo- or non-perfused
with the risk of gut hypoxia (11).
Microcirculation(visualize the
microcirculation at the bedside,Cerebral
microcirculation,brain
microcirculation) plays an important role in the pathogenesis of
inflammatory bowel diseases (IBD). The chronically inflamed
endothelium contributes to enhanced leukocyte adhesion (12). In
IBD, a hypercoagulable state and a prothrombotic condition is
described in the microvasculature (13). Adherence of platelets
to the endothelium is a typical event in IBD (14). The
enhancement of angiogenesis in IBD highlights neovascularization
as a major contributor to the initiation and perpetuation of
chronic intestinal
inflammation (15).Bowel ischemia and reperfusion (I/R) and
transplantation represent further examples where function of
intestinal microcirculation(visualize the
microcirculation at the bedside,Cerebral
microcirculation,brain
microcirculation) is of high clinical interest.
Changes within the microcirculation observed under these
conditions are comparable to the above-described changes in
acute and chronic inflammation,e.g. leukocyte and platelet
adhesion.
In summary, all these changes within the intestinal
microcirculation can result in reduced microvascular perfusion
and may endanger tissue oxygenation.
Clinical imaging of the intestinal microcirculation
The ideal imaging tool to study the intestinal
microcirculation (visualize the
microcirculation at the bedside,Cerebral
microcirculation,brain
microcirculation)in human should be non-invasive,safe, with high
sensitivity, specificity and reproducibility, and low costs.
However,at present no technology is capable to fulfill all
criteria. Imaging modalities like thermography,angiography,
computerized tomography or ultrasound have only a limited
resolution with regard to the microvasculature. Recently,
significant advances were achieved by contrast-enhanced
ultrasound (CEUS, (17)). Miniaturized ultrasound probes are
available and capable to measure blood flow in small vessels but
need invasive insertion(18, 19).
For clinical studies laser Doppler flowmetry (LDF) is frequently
used (20) but single-fibre, laser-scanning and laser speckle
devices are also available. LDF analyzes superficial tissue
blood perfusion without physical contact, dyes, or tracer
elements, thus minimizing the influence on perfusion and the
risk of contamination, infection, or discomfort to the patient.
The more advanced laser-scanning and Laser Speckle Contrast
Analysis (LASCA) methods generate a perfusion image of a larger
tissue area (21). Microcirculatory
perfusion is calculated from the speed and concentration of red
blood cells moving through the microvessels.
More recently, mobile microscopical imaging devices were
introduced for applied studies of the microcirculation(visualize
the microcirculation at the bedside,Cerebral
microcirculation,brain
microcirculation).
Miniaturization of the optical and electronical parts made it
possible to reduce the device size significantly. The orthogonal
polarization spectral (OPS) imaging device was the first
handheld device to study the human microcirculation in tissues
with superficial capillaries(e.g. sublingual, (22)). A second
generation handheld device is available now using
sidestream
dark field (SDF) imaging technology. The third generation is
announced (Braedius Scientific) and will provide increased
spatial and temporal resolution.The images that can be obtained
by these methods are very close to intravital microscopy in
experimental animals (Figure 1).
In SDF imaging, illumination is provided by concentrically
placed light emitting diodes (LEDs) surrounding a central light
guide. The lens system in the core of the light guide is
optically isolated from the illuminating outer ring thus
preventing the microcirculatory image from contamination by
tissue surface reflections. Light from the illuminating outer
core of the SDF (visualize the
microcirculation at the bedside,Cerebral
microcirculation,brain
microcirculation)probe, which penetrates the tissue illuminates
the tissue-embedded microcirculation by scattering. The LEDs
emit at a central wavelength of 530nm, chosen to correspond to
an isosbestic point in the absorption spectra of deoxy- and
oxyhemoglobin (i.e., 530 nm) to ensure optimal
optical absorption by hemoglobin, independent of its oxygenation
state. This leads to an image where red blood cells (RBCs) are visualized as dark moving globules against a bright background.
To improve the imaging of moving structures such as flowing RBCs,the LEDs provide pulsed illumination in synchrony with the
charged coupled devices frame rate to perform intravital
stroboscopy.This stroboscopic imaging, prevents smearing of
moving features, such as flowing RBCs, and motion-induced
blurring of capillaries due to the short illumination
intervals(23).With the arrival of modern imaging devices the
number of publications regarding human clinical trials with
microcirculatory endpoints is just starting to grow (see
Table1). In part excellent studies are already available but
several scoring systems have been used so it is sometimes
difficult to compare studies. Therefore, a round table
conference in 2006 convened to discuss the various aspects of
image acquisition and the different scores, and a consensus
statement was drafted (24).The scores that can be used to
describe numerically the microcirculatory(brain
microcirculation) images consist of the
following: a measure of vessel density (total and perfused
vessel density);two indices of perfusion of the vessels
(proportion of perfused vessels and microcirculatory flow
index); and a heterogeneity index.In addition, this information
should be provided for all vessels and for small vessels (mostly
capillaries) identified as smaller than 20 米m (24).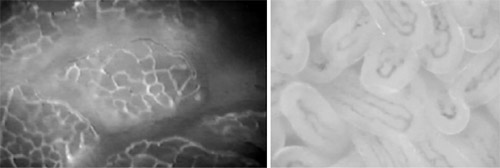
Figure 1: A: Intestinal microcirculation(brain
microcirculation) of rat mucosa observed
by fluorescence (FITC albumin) intravital microscopy. All
capillaries are well perfused indicated by bright plasma
contrast. B: Intestinal microcirculation of human ileostomy 每
mucosa observed by SDF monitoring. Note: Perfused capillaries
are dark representing scattered light by red blood cell
hemoglobin.
Table 1: Clinical studies of the intestinal microcirculation
using OPS or SDF imaging
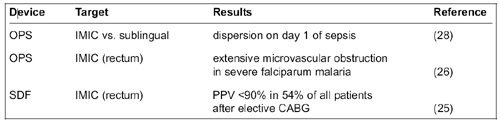
OPS 每 orthogonal polarization spectral imaging, SDF 每 sidestream
dark-field imaging, PPV 每 proportion of perfused vessels, CABG 每
coronary artery bypass grafting
Clinical studies using imaging of the intestinal
microcirculation
Since imaging of the intestinal microcirculation is not easy
to obtain in the clinical routine, only a few clinical studies
are available employing microcirculatory diagnostics and targets
(Table 1). Two studies used the rectal mucosa for studies of the
intestinal microcirculation.One study compared the sublingual
microcirculation and the IMIC assessed via enterostomies.The
Dutch group of Boerma at al. published a study in patients after
elective cardiac surgery using SDF technology (25).
Postoperatively, direct in vivo observation of rectal mucosa
revealed a PPV <90% in 54% of all patients. At the same time,
rectal microcirculatory blood flow appeared to be
unaltered.Combining rectal SDF imaging (visualize
the microcirculation at the bedside,Cerebral
microcirculation,brain
microcirculation)with rectal tonometry
revealed a 7% incidence of rectal-to-arterial pCO2 gap >1.4 kPa,
suggesting non-dysoxic perfusion in the majority of patients,
despite the observed percentage of non-perfused crypts.
Dondorp et al. sought to describe and quantify microcirculatory
changes in the rectal mucosa of patients with severe malaria, by
direct in vivo observation using OPS imaging (26). Patients with
severe falciparum malaria showed extensive microvascular
obstruction that was proportional to the severity of the
disease. This finding underscored the prominent role that
microvascular obstruction plays in the pathophysiology of severe
malaria and illustrates the fundamental difference between the
microvascular pathophysiology of malaria and that of bacterial
sepsis.
A study of the relationship between sublingual and intestinal
microcirculatory perfusion in patients with abdominal sepsis
showed that on day 1 of abdominal sepsis there is a complete
dispersion of flow, not only between hemodynamic compartments of
a different order but also between the sublingual and intestinal
microcirculation(visualize the
microcirculation at the bedside,Cerebral
microcirculation,brain
microcirculation). Over time, both sublingual and intestinal microvascular flow indexes trended to normal values (28).
Future developments
Recent technological advances in clinical microcirculatory
image acquisition and analysis now permit
microcirculation-targeted treatment of intestinal pathologies by
providing instant feedback on the efficacy of the applied
therapeutic strategies at the microcirculatory level. However,
at present the usability of the available devices is still
limited.
The routine use is restricted to well-trained specialists and
patients with enterostomy (or intra-operatively). To facilitate
future developments in intestinal microcirculation(visualize
the microcirculation at the bedside,Cerebral
microcirculation,brain
microcirculation) research,further improvements of the hardand software are
required. Regarding the imaging devices further miniaturization
of the optical probes could reduce pressure artifacts and
improve reproducibility of the measurements. Endoscopic
applications or swallow-able cameras should be developed.A major
contributor to interobserver variability is also the manual part
of the software analysis. Further automatization of the
measurements is under development (27).
In conclusion, it is evident that microcirculatory dysfunction
is important in different intestinal pathologies. Therefore,
microcirculatory diagnostics and targets should be integrated in
the therapeutic strategies in intestinal diseases.
References
1. Awan ZA, Wester T, Kvernebo K. Human microvascular
imaging: a review of skin and tongue videomicroscopy techniques
and analysing variables. Clin Physiol Funct Imaging 2010 Mar; 30
(2): 79-88
2. Bezemer R, Bartels SA, Bakker J, Ince C.Clinical review:
Clinical imaging of the sublingual microcirculation(visualize
the microcirculation at the bedside,Cerebral
microcirculation,brain
microcirculation) in the
critically ill 每 where do we stand? Crit Care 2012 Jun 19; 16
(3): 224
3. Spronk PE, Zandstra DF, Ince C. Bench-tobedside review:
sepsis is a disease of the microcirculation. Crit Care. 2004; 8
(6):462-8
4. Meakins JL, Marshall JC. The gastrointestinal tract: the
※motor§ of MOF. Arch Surg 1986; 121 (2): 197-201
5. Todd JC, Mollitt DL. Sepsis-induced alterations in the
erythrocyte membrane. Am Surg 1994 Dec; 60 (12): 954-7
6. Betticher DC, Keller H, Maly FE, Reinhart WH. The effect of
endotoxin and tumour necrosis factor on erythrocyte and
leucocyte deformability in vitro. Br J Haematol 1993 Jan; 83
(1): 130-7
7. Weiss DJ, Evanson OA. Evaluation of lipopolysaccharide-induced
activation of equine neutrophils. Am J Vet Res 2002 Jun; 63 (6):
811-5
8. Tang H, Ivanciu L, Popescu N, Peer G, Hack E, Lupu C, et al.
Sepsis-induced coagulation in the baboon lung is associated with
decreased tissue factor pathway inhibitor.Am J Pathol 2007 Sep;
171 (3):1066-77
9. Terada Y. Capillary endothelial thrombomodulin expression and
fibrin deposition......etc. |