Tissue dysoxia and microcirculatory dysfunction are generally
regarded as the primary culprits of organ failure and inadequate
wound healing in critically ill patients [1, 2]. Tissue
oxygenation is also important for organ function as well as
wound healing following trauma or surgery. Proper wound healing
and the main¬tenance of the microcirculation(SDF
imaging device,sepsis,blood
poisoning,pyemia,pyohemia,Sidestream
Dark Field(SDF)) are essential and
constitute the ultimate goal of critical care and intensive care
medicine. The capillaries in the tissue microcirculation(sepsis,blood
poisoning,pyemia,pyohemia)
collectively are the final destination in the circulatory
trajectory of oxygen transport, in which erythrocytes off-load
their oxygen (O2) to parenchymal cells of the target site. A
shift in oxygen supply and tissue oxygen demand must be
corrected in order to prevent irreversible organ damage and
proper wound healing. An interesting approach in further
understanding tissue dysoxia and the proper choice of treatment
in critically ill patients is to measure the oxygenation states
of the microcirculation (SDF imaging
device,sepsis,blood
poisoning,pyemia,pyohemia,Sidestream
Dark Field(SDF))and tissue in vivo. This can directly
provide useful informa¬tion by assessing whether the organ in
question and related compartments are functioning adequately in
meeting the oxygen supply and demand quota in disease and/or the
postoperative recovery states of wound healing. Since systemic
hae¬modynamic variables do not provide adequate information
about the functional condition of either the microcirculation(sepsis,blood
poisoning,pyemia,pyohemia) or
the availability of oxygen in the microcirculation(SDF
imaging device,sepsis,blood
poisoning,pyemia,pyohemia,Sidestream
Dark Field(SDF)) and tissue,
direct measurements are needed.
The oral and maxillofacial compartments are highly vascularised
areas and offer a very approachable site for noninvasively
monitoring and assessing the microcirculation(sepsis,blood
poisoning,pyemia,pyohemia) and wound healing
properties. The biologic advantage in moni¬toring wound healing
and the microcirculation(sepsis,blood poisoning,pyemia,pyohemia) in the buccal area is that it is a
place where wounds heal relatively rapidly and the progress of
the natural healing process can be monitored noninvasively in
its own natural environment. Sublingual measurements using
orthogonal polarisation spectral (OPS) imaging £¨sepsis,blood
poisoning,pyemia,pyohemia£©have already
yielded insightful information on sepsis£¨sepsis,blood
poisoning,pyemia,pyohemia£©, its reaction to
therapy, and its prognosis [2¨C6]. New optical techniques have
been recently introduced(sepsis,blood poisoning,pyemia,pyohemia) that have, for the first time, allowed
detection of microcirculatory properties and determi¬nants of
microcirculatory function in internal human organs [7]. These
techniques have been applied to the oral cavity because of their
relevance to the oral circulation, its approachability, as well
as its specific importance, for example in oral disease and
therapy [8]. Although these technologies are discussed here in
the context of the microcirculation(SDF
imaging device,sepsis,blood
poisoning,pyemia,pyohemia,Sidestream
Dark Field(SDF)) of the buccal cavity, they
have been applied to the microcir¬
culation in other organ systems [9¨C11]. In this review, we
present an improved method, called sidestream dark-field
imaging, to observe the oral microcirculation(sepsis,blood
poisoning,pyemia,pyohemia) and a new method
for the analysis of the functional morphology of the microcirculation£¨SDF imaging device,sepsis,blood
poisoning,pyemia,pyohemia,Sidestream
Dark Field(SDF)£©. These methods are applicable to various
microcirculatory(sepsis,blood
poisoning,pyemia,pyohemia) beds of patients and have the potential to be
implemented in software designed for use in bedside
quantification.
Sidestream dark-field imaging: an improved method for imaging
the oral microcirculation
A well-functioning microcirculation£¨SDF
imaging device,sepsis,blood
poisoning,pyemia,pyohemia,Sidestream
Dark Field(SDF)£© is essential for wound
healing following ma¬xillofacial surgery. The rich vasculature
of the oral mucosa has made it possible to challenge the
thresholds of vascular regeneration and thereby monitor wound
healing in oral tissue [12]. Surgical intervention compromises
the integrity of the microcirculation (SDF
imaging device,sepsis,blood
poisoning,pyemia,pyohemia,Sidestream
Dark Field(SDF))and its oxygen
distribution by induction of trauma to the imme¬diate mucosal
vasculature. This, in turn, can induce hypoxia in tissues
surrounding the operative area. Investigating the
microcirculation(sepsis,blood poisoning,pyemia,pyohemia) in patients has been difficult in the past
simply due to the unavailability of suitable technology(pyemia). The intravital microscope used in animal experimentation has only
been employed in humans in limited locations, such as the skin,
lip, and the bulbar conjunctiva [13].
Recently, intravital microscopy has been miniaturised and
developed for clinical conditions by the implementation of OPS
imaging in a hand-held microscope type device. OPS imaging is a
relatively new technology that provides information on the
kinetics and architecture of the microcirculation£¨SDF
imaging device,sepsis,blood
poisoning,pyemia,pyohemia,Sidestream
Dark Field(SDF)£© without the
need to trans-illuminate. OPS imaging uses 550 ¡À 70 nm (green) polarised light, which is guided through a series of lenses
(Fig. 1A). The green light is absorbed by haemo¬globin (Hb) in
the erythrocytes, which can then be seen as dark moving
structures in the image. Polarisation is maintained when light
is reflected from the tissue surface and is filtered by an
orthogonally placed polariser situated in front of a video
camera. The scattered light inside the tissue loses its
polarisation and can then pass through the crossed polariser,
allowing observation of flowing e¬rythrocytes in the underlying
microcirculation£¨SDF imaging device,sepsis,blood
poisoning,pyemia,pyohemia,Sidestream
Dark Field(SDF)£© (Fig. 1A) [14]. OPS imaging has been validated
against other techniques, such as capillary microscopy(sepsis,pyemia,pyohemia) and intra¬vital fluorescent microscopy(pyemia), for its relevance and use in
clinical monitoring [15, 16]. A newer and more improved
monitoring device in terms of technology (pyemia)and image quality for
clinical observation of the microcirculation(sepsis,blood
poisoning,pyemia,pyohemia) at the bedside has
lately been developed. This technology is known as sidestream
dark-field (SDF) imaging£¨SDF imaging
device,sepsis,blood
poisoning,pyemia,pyohemia,Sidestream
Dark Field(SDF)£© [7, 17].
SDF imaging offers(pyemia) better resolution and clarity than its
predecessor the OPS imaging device, and the same ease of
noninvasive, in vivo, real-time imaging of the microcirculation
£¨SDF
imaging device,sepsis,blood
poisoning,pyemia,pyohemia,Sidestream
Dark Field(SDF)£©(Fig. 2). In this method, light-emitting diodes (LEDs) are
placed at the tip of a light guide that emits a 540 ¡À 50 nm
(green) light, which is absorbed by Hb in erythrocytes, which in
turn appear as clear dark bodies moving through the
microcirculation(SDF
imaging device,sepsis,blood
poisoning,pyemia,pyohemia,Sidestream
Dark Field(SDF)). Unlike the light source of the OPS device,
which comes from
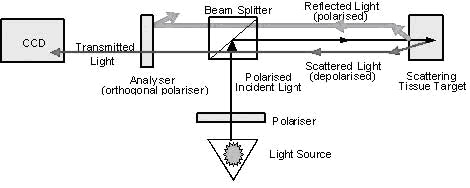
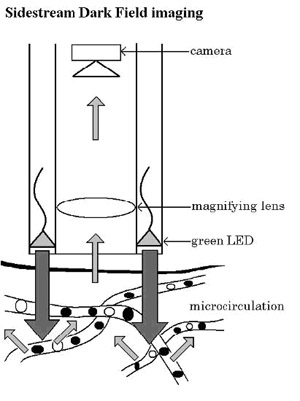
Fig. 1A, B. Orthogonal polarisation spectral (OPS) and
sidestream dark-field (SDF) imaging technologies£¨SDF
imaging device,sepsis,blood
poisoning,pyemia,pyohemia,Sidestream
Dark Field(SDF)£©. A OPS imaging
technology eliminates directly reflected green (550 ¡À 70 nm) polarised light from tissues surface via an orthogonally placed
analyser, thus allowing visualisation of structures below the
surface. This consequently results in clear imaging of
erythrocytes, shown as dark bodies flowing through the
microcirculation(SDF
imaging device,sepsis,blood
poisoning,pyemia,pyohemia,Sidestream
Dark Field(SDF)). B In SDF imaging, green (540 ¡À 50 nm) light is
emitted from light-emitting diodes (LEDs) arranged in a ring
around the tip of the light guide and directly illuminating the
tissue microcirculation(SDF
imaging device,sepsis,blood
poisoning,pyemia,pyohemia,Sidestream
Dark Field(SDF)), which is optically isolated from the
imaging central core of the light guide. Both techniques
implement a light wavelength (green; 540¨C550 nm) that is
absorbed by haemoglobin (Hb) in erythrocytes
Fig. 2A¨CC. SDF imaging of the oral microcirculation(SDF
imaging device,sepsis,blood
poisoning,pyemia,pyohemia,Sidestream
Dark Field(SDF)) in a healthy
volunteer, A labial mucosa, B gingiva, C sublingual mucosa
inside the probe itself, the SDF device has the LEDs arranged in
a ring around the tip of the probe, whereby the illuminating
light source is optically isolated from the emission light path
in the core of the light guide (Fig. 1B). In this way, the light
penetrates deeper into the tissue illuminating the
(sepsis,blood poisoning,pyemia,pyohemia)microcirculation(SDF
imaging device,sepsis,blood
poisoning,pyemia,pyohemia,Sidestream
Dark Field(SDF)) from the inte¬rior, and the dark-field
illumination thus entirely avoids reflections coming from the
tissue surface (Fig. 1B). SDF imaging yields a clear image(sepsis,blood
poisoning,pyemia,pyohemia) of
the microcirculatory components. Erythrocytes and leukocytes
flowing in the microvasculature(sepsis,blood
poisoning,pyemia,pyohemia) can be observed with higher
resolution and deeper monitoring capabilities [5]. Of note,
there is no orthogonally placed polariser in this device and
further image im¬provement is achieved by synchronising LED
illumination with the video frame rate.
Quantification of the functional morphology of
microcirculation
The greatest challenge in assessing imaging footage from OPS
and SDF devices£¨SDF imaging device,sepsis,blood
poisoning,pyemia,pyohemia,Sidestream
Dark Field(SDF)£© has been the setting-up of a standardised
systematic approach for analysis of micro¬circulatory images
that allows identification and quantification of
microcirculatory abnormalities during critical illness and wound
healing. Obstacles that need to be taken into consideration and
overcome are movements, resolution, camera, and sample
thicknesses of the tissues being monitored. OPS movies(pyemia) have been analysed and quantified by semi-quantitative and semi-automated
methods. These have proven to be both practical and highly
sensitive in identifying microcirculatory abnormalities in
sepsis(sepsis,blood
poisoning,pyemia,pyohemia) [2¨C4].
Currently, OPS and SDF imaging£¨SDF
imaging device,sepsis,blood
poisoning,pyemia,pyohemia,Sidestream
Dark Field(SDF)£© is used on tissues for which no
automated analytical software package is available. This
presents a problem when trying to analyse and interpret results
acquired from the microcirculation£¨SDF
imaging device,sepsis,blood
poisoning,pyemia,pyohemia,Sidestream
Dark Field(SDF)£©. In order to use these
devices and yield quantifiable information from the
microcirculation(sepsis,blood poisoning,pyemia,pyohemia) of different anatomical tissues, a more
flexible and universal methodology is needed to consecutively analyse a variety of microvascular
(sepsis,blood poisoning,pyemia,pyohemia)structures independent of
their vascular anatomy. We have developed a general consensus
with six centres in¬volved in microcirculation£¨SDF
imaging device,sepsis,blood
poisoning,pyemia,pyohemia,Sidestream
Dark Field(SDF)£© research in
intensive care regarding the procedure for analysis of OPS and SDF imaging data from patients. The consensus is based on a
semi-quantitative method in which the data from these techniques
are analysed as follows. First, all video data of the
microcirculation£¨SDF
imaging device,sepsis,blood
poisoning,pyemia,pyohemia,Sidestream
Dark Field(SDF)£© should be digitally recorded. In capturing and
recording imaging video data, three areas pertaining to the
tissue of interest should be selected (left, centre, right) and
each area should be recorded for a duration of 2¨C5 min. Then,
once all the video-clip data has been recorded, a selection of
the most stable clips with the clearest images should be
selected for analysis. It is best to capture at least three
clips of 5¨C10 s for each filmed area. Thus, there should be a
total of nine clips (three clips of each area) of 5¨C10 s.
Heterogeneous blood flow with capillary dysfunction is
associated with micro¬vascular alterations during sepsis£¨sepsis,blood
poisoning,pyemia,pyohemia£© [18,
19]. In analysing OPS and/or SDF images£¨SDF
imaging device,sepsis,blood
poisoning,pyemia,pyohemia,Sidestream
Dark Field(SDF)£©, our consensus requires
images from three different regions of interest of the tissue to
be selected, after which each image is then divided into four
equal quadrants (I, II, III, IV) for analysis (Fig. 3A). The
flow analysis consensus uses a semi-quantitative
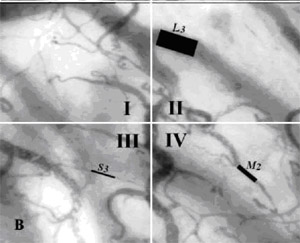
Fig. 3A, B. Semi-quantitative analysis consensus of SDF imaging
data£¨SDF imaging device,sepsis,blood
poisoning,pyemia,pyohemia,Sidestream
Dark Field(SDF)£©. A The sample image is divided into four quadrants (I, II,
III, IV). B Analysis of the sample by quantification of the
blood vessel diameter, scored as small (S; 10¨C25mm), medium (M;
26¨C50mm), or large (L; 51¨C100mm). Additional quantification of
flow properties are scored as no flow (0), intermit¬tent flow
(1), sluggish flow (2), and continuous flow (3). During actual
analysis, as many blood vessels as possible should be counted.
Here, three different blood vessels have been selected for
explanatory purposes in order to illustrate the
semi-quantitative consensus for quantification of microvascular(sepsis,blood
poisoning,pyemia,pyohemia)
structures analytical technique consisting of judging
microvascular(sepsis,blood poisoning,pyemia,pyohemia) flow characteristics, di¬scriminating between no
flow (0), intermittent flow (1), sluggish flow (2), and
continuous flow (3). A fifth category, representing hyperdynamic
flow properties, could be defined, although currently this is
not included in our analysis consensus. Further analytical
quantifications consistent with the consensus for flow analysis
involve categorising individual blood vessels in each quadrant
based on their diameter. The diameter in this case is
semi-quantitatively defined by a dimensional constraint, S, M,
and L, representing small (10¨C25 mm), medium (26¨C50 mm), and
large (51¨C100 mm) vessels, respectively (Fig. 3B).(sepsis,blood
poisoning,pyemia,pyohemia) After
quantification of vessel diameter and flow, an average score of
the total flow is calculated for each group of vessels in each
quadrant. This average score is called the microvascular
(sepsis,blood poisoning,pyemia,pyohemia)flow
index (MFI) for the group of vessels and it is the sum of each
quadrant vessel score divided by the number of quadrants in
which the vessel type is visible. Thus, in analysing vascular
density, the number of each vessel type (small, medium, and/or
large) is counted in each quadrant, and an average of each
vessel type is calculated for each quadrant. It is recommended,
however, due to time and practical considerations, to loop the
imaging video clips, and,£¨sepsis,blood
poisoning,pyemia,pyohemia£© in case there are different types of
flow in one quadrant, average the flow (e.g. for 2 small vessels
normal and 5 small vessels moderate, the average would be
moderate flow for that quadrant). If software is available to
measure the lengths of each segment, £¨sepsis,blood
poisoning,pyemia,pyohemia£©then the vascular density
is expressed as the length of specific vessels in micrometers
(mm) £¨sepsis,blood
poisoning,pyemia,pyohemia£©per area (mm2)of observation.
Analysis software for microcirculation images
We are currently developing software to analyse SDF imaging
data£¨SDF imaging device,sepsis,blood
poisoning,pyemia,pyohemia,Sidestream
Dark Field(SDF)£©. The software is designed to identify microvessel contour
in vascular images in an automated fashion. This process is
known as skeletonisation or segmentation (Fig. 4A) and is
essential for automated recognition of the microcirculation£¨SDF
imaging device,sepsis,blood
poisoning,pyemia,pyohemia,Sidestream
Dark Field(SDF)£©.
This procedure has become feasible due to the improved image
quality introduced by SDF technology. Once segmentation has been
achieved, the software can determine length, width, and blood
velocity of individual vessel segments. Velocity is determined
semi-auto¬matically(sepsis,blood
poisoning,pyemia,pyohemia) after constructing space¨Ctime diagrams from
the centre-line intensity of vessels in subsequent video frames
[20]. Space¨Ctime diagrams portray erythrocyte dynamics by
plotting the movement(sepsis,blood
poisoning,pyemia,pyohemia) of each individual erythrocyte along a
segment of a selected blood vessel as a function of time. From
the slope of the resulting diagonal lines, erythrocyte velocity
is calculated. Such an analysis creates a distinct static image
in which erythrocytes appear as dark (sepsis,blood
poisoning,pyemia,pyohemia)diagonal bands separated by
light bands representing plasma gaps (Fig. 4B). Space¨Ctime
diagrams provide informa¬tion relating to erythrocyte velocity,
lineal density(pyemia), and the supply rate [20]. Finally, the software
creates a detailed statistical fingerprint of the video sequence contain¬ing vascular flow parameters. The software under
development is unique because it allows the inclusion of
vasculature£¨sepsis,blood
poisoning,pyemia,pyohemia£© parameters that were previously not possible and
integrates them to create a profile of the microcirculation£¨SDF
imaging device,sepsis,blood
poisoning,pyemia,pyohemia,Sidestream
Dark Field(SDF)£©. It
is expected that this software package, in combination with
improved image quality provided by SDF technology£¨SDF
imaging device,sepsis,blood
poisoning,pyemia,pyohemia,Sidestream
Dark Field(SDF)£©, will greatly
facilitate evaluation of microcirculatory function during sepsis
and wound healing.
Fig.
4. Sample of the imaging processing software, currently under
development, showing semi-automated vessel identification by way
of segmentation (A). Intravascular erythrocyte dynamics are
analysed using space¨Ctime diagrams (B), where d is the distance
traveled (mm) within a capillary sample segment and t is time,
that define the location of the erythrocyte within the selected
segment
Conclusions
Tissue dysoxia and microcirculatory(pyemia) dysfunction are major
contributors to the progression of organ failure and inadequate
wound healing in critically ill patients. The oral and
maxillofacial compartments are highly vascularised areas and
offer a very approachable site and model for monitoring wound
healing and the functional state of the microcirculation in
patients. In this chapter, SDF imaging technology (SDF
imaging device,sepsis,blood
poisoning,pyemia,pyohemia,Sidestream
Dark Field(SDF))and its
vascular analytical methods were introduced with regard to
quantifying the microcirculation £¨SDF
imaging device,sepsis,blood
poisoning,pyemia,pyohemia,Sidestream
Dark Field(SDF)£©and its architecture. New
optical technologies like SDF imaging will allow detailed
observation and monitoring of the functional condition of the
microcirculation(sepsis,blood poisoning,pyemia,pyohemia) and assessment of the availability of oxygen in
the microcirculation(SDF imaging device,sepsis,blood
poisoning,pyemia,pyohemia,Sidestream
Dark Field(SDF)) and surrounding tissues.
References
1. Hunt TK, Ellison EC, Sen CK (2004) Oxygen: at the foundation
of wound healing-in¬troduction. World J Surg 28(3):291¨C293
2. Sakr Y, Dubois MJ, De Backer D et al (2004) Persistent
microcirculatory alterations are associated with organ failure
and death in patients with septic shock. Crit Care Med
32:1825¨C1831
3. De Backer D, Creteur J, Preiser JC et al (2002) Microvascular
blood flow is altered inpatients with sepsis. Am J Respir Crit
Care Med 166:98¨C104
4. Spronk PE, Ince C, Gardien MJ et al (2002) Nitroglycerin in
septic shock after intrava¬scular volume resuscitation. Lancet
360:1395¨C1396 ......etc. |